Authors: Judi Fusco & Patricia Schank
Printer-Friendly PDF | Google Doc for Comments | Questions? Contact CIRCL.
Overview
Educators, companies, and governments around the world share the desire to support and engage underrepresented groups in science and engineering. Some programs have had success in the goal of increasing the interest and engagement of underrepresented groups in engineering and STEM. Many of these developed curriculum, intensive teacher training, student competitions, and other practices––such as providing mentors and same-gender/race role models, and using real motivating problems in the students’ community––to engage underrepresented groups in engineering and design. In general, the most successful programs that have an impact on underrepresented groups are those that have role models that youth can identify with, and that develop appropriate situational, culturally relevant interactions with target youth (McGill, Decker, & Settle, 2015).
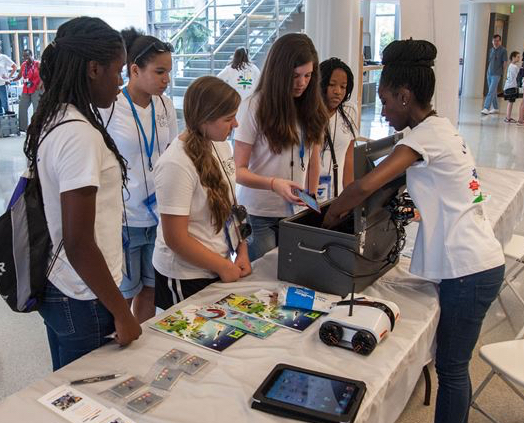
Still, large disparities persist in innovation rates by socioeconomic class, race, and gender––and the gap is not explained by innate ability (e.g., early childhood test scores; Bell et al., 2017). Consider the College Board’s Advanced Placement Program, which is one gateway to the STEM career pathway. While the number of high school students taking the AP Computer Science exam has been increasing annually, and the number of exam takers in 2016 rose 17%, female, Latinx, and African American participation remains low; just 23% of exam takers were female, 11.5% were Latinx, and 3.7% were African American (Ericson, 2017; Hinton, 2016). In 8 states, fewer than 10 girls took the exam, and in 2 states, no girls took the exam. And even when women, girls, and minorities enter a STEM career pathway, they leave at a higher rate––at multiple points––from middle school to community college to even tenured faculty in STEM (Burke & Mattis, 2007; Griffith, 2010). Margolis & Fischer (2003) interviewed women who were dropping out of computer science in college and found that professors favored men who had more experience in CS. From a social justice perspective (Bienkowski, 2018), even when anyone can take advantage of an opportunity (equality), not everyone comes to that opportunity prepared in the same way or experiences that opportunity the same way (equity).
Why worry about diversity in computing and engineering? Disparity in opportunity is not fair to individuals, economically and educationally, and society needs everyone’s perspectives and contributions to solve important problems. Inclusion is critical for innovation: diversity increases innovation (e.g., patents), partly because a variety of perspectives lead to new ideas, earlier identification of problems, and more effective science (Forbes Insights, 2011; Medin & Bang, 2014; Bienkowski, 2018). Bell and colleagues (2017) argue that if women, minorities, and children from low-income families were to invent at the same rate as white men from high-income families, the rate of innovation in America would quadruple; “There are many “lost Einsteins” – people who would have had highly impactful inventions had they been exposed to careers in innovation as children” (p. 1)––and had they been given continued opportunities, role models, mentors, and other support provided to those who are already highly rewarded.
Advancing educational, economic, and innovative opportunity requires special attention to issues that prevent equitable participation (Blikstein, 2018). Governments and funding agencies have responded with several initiatives––including NSF’s CS4All and STEM+C programs. Expanding access to computer science has been announced as a priority by 40 states (Code.org, 2017), 27 states have enacted computer science curricula for their K-12 public schools, and 13 more are in the process of developing statewide CS standards (Blikstein, 2018).
This primer reviews practices from selected work that that has helped broaden youth participation in computer science and engineering (see Key Lessons). These practices were drawn from the literature and from a review of selected successful programs (see Projects). We also discuss deeper issues of identity, interest, and self-efficacy that can hold youth back, and ways to impact youth interest and desire to pursue STEM and engineering careers.
Key Lessons
Mentors help youth develop interest, identity, and self-efficacy. As learners develop, they need others to help them. Teacher often play this role through formal instruction. Mentors use many of the same practices as good teachers, but mentoring relationships are often more informal and focused on offering advice from a perspective of experience. Mentors can help youth development their identity by promoting an interest, and over time, reshaping beliefs about an area of interest (e.g., seeing themselves as someone who could work in the area). A young person who has a mentor in computer science typically engages in more computer science education and also has a more diverse set of beliefs around who can be a computer scientist (Ko & Davis, 2017). Ko and Davis developed a 6-week course in which the teacher explicitly created mentoring relationships with each student that included a personal interest in their trajectory of learning (e.g., talking with them everyday, having end of the course mentorship conversation, and offering email support after the course ended). After the course, there was a significant increase in student interest in computing for students who had a mentor; the mentorship effect was more powerful than gender or socioeconomic status.
Youth need engineering role models who reflect their identities and interests. A role model is an image of someone admired, someone who an individual aspires to be like. Unlike a mentor or teacher, a role model may not play a direct role in the success of the individual. However, role models still provide inspiration and motivation. For example, girls are more likely to become inventors in a field if they simply grow up in an area with more female inventors (but not male inventors) in that field (Bell et al., 2017). In computer science specifically, having women as role models and mentors increases the likelihood that girls will pursue the field (Wang et al., 2015). Men can also serve as successful mentors and role models for girls if they do not conform to male computer science stereotypes (Cheryan et al., 2011). Diversifying the image of computer scientists and engineers — and having teachers, facilitators, and peers who push back against stereotypes — increases youth’s sense of belonging and interest in the field (Ruiz, 2017; Cheryan, Master, & Meltzoff, 2015).
Engaging existing relationships in youths’ lives helps increase youth success. Youth express positive attitudes toward science when they experience success and receive support from important people in their lives at home, in school, and in their communities (Aschbacher, Li, & Roth, 2010). Family plays a critical role in encouraging youth and exposing them to opportunities, and it’s especially helpful to engage parents in the effort to increase youth participation in computer science and related fields (Wang, Hong, Ravitz, Ivory, 2015). It is also helpful for educators to discuss with youth how STEM professionals think about people’s needs while they work, and what the student could uniquely contribute if they were to pursue computer science or engineering. An exciting curriculum that youth connect with––like Hour of Code or attending a computer camp––can also be a “triggering event” that helps youth develop an initial interest in the subject (Ko & Davis, 2017).
Focus on real world interests to attract underrepresented youth. “Powerful learning experiences result when students have the opportunity to connect their interests from outside of school to learning opportunities in more academic contexts” (Reich & Mizuko, 2017). For girls and non-dominant youth, it’s important to create entry points that support diverse interests (e.g., fashion, sports, dance, health). Margolis, Fisher, and Miller (1999) report that when asked why they are taking computer science, undergraduate males typically report interest in computers while about half of females describe their interest as it relates to areas of interest such as health, education, science, or art. Girls may be more interested in careers in health and medicine than engineering (Sadler et al., 2012). These interests provide an opportunity to highlight new pathways into engineering: many medical careers (such as biomedical engineers) work at the intersection of engineering, life sciences and medicine, so they need computer science background. McGee and Bentley (2017) report that African-American and Latinx students are more likely to want to go into science because of their interest in social justice and improvements to the social world that science could facilitate. Tapping into interests that different groups have is important for success.
Facilitate an extended, supported experience to enable success. As mentioned earlier, youth persist when they receive support and experience success (Aschbacher, Li, & Roth, 2010). In fact, if youth have a bad first experience with computing, it can turn them off completely (Ko, 2017): “Research shows over and over that most learners, people of all ages, start with low programming self-efficacy, and that without early, repeated successes in writing programs, this self-efficacy is quickly exhausted, causing youth to give up… [which] may lead to reduced interest in coding and lower programming self-efficacy. Female learners in particular are prone to internalizing these failures into their identity.” It’s best to ease youth into coding so they have a successful experience.
Issues
Lack of opportunities and connections explain part of the lower engagement of underrepresented groups in STEM and engineering. Even when opportunities are made available, deeper issues of identity, interest, and self-efficacy continue to hold youth back.
Identity is both an important part of, and a way to discuss, learning. Identity is more than an individual’s beliefs about him or herself; it includes not only how you think of yourself but also your perception of how others see you and what is promoted by society as acceptable or desirable. From ambient messages in the culture, individuals perceive what others think of them and who is typically identified a certain way––for example, who is and can be an engineer. Identity surfaces as one way of understanding why we see robust underrepresentation of particular groups. People make important decisions about their future and how they engage in the pursuit of their careers based on identity. In many different ways, learners can feel marginalized or encouraged because of their identity. Developing learning environments that integrate learner interests, are sensitive to identity, and encourage all learners to be participants and take on new identities––especially in STEM fields––is challenging and needed work (Bell, Van Horne, Cheng, 2017). To develop STEM identities, young people need access to sustained, high quality experiences, and, particularly in the early adolescent years, they need to see others like themselves as role models.
Interest is a related component necessary to develop future STEM workers. Hidi and Renninger (2006) define interest as a person’s heightened affect and predisposition towards a subject depending on their knowledge, feelings towards it, and value of it. Interest goes from an initial spark that requires extrinsic motivators to keep it going, to well-developed when it does not require extrinsic motivators. Of course, a learner will always benefit from external rewards or recognition around their interests, but a learner with well-developed interests in a topic will stay involved even without rewards or requirements. Ko and Davis (2017) discuss “triggering events” as important to helping develop an interest in computing, but that more is needed to develop it and sustain it. Hidi and Renninger (2006) describe interest development in a 4 phase model. At a high level, the 4 phases can be described as follows:
- Triggered Situational Interest––sparked by events, personally relevant experiences, and novel or surprising events. Usually a precursor to further development.
- Maintained Situational Interest-–sustained by meaningful tasks, and deeply engaging, personally relevant instructional projects can help this develop.
- Emerging Individual Interest––marked by repeated engagement, positive affect, going beyond the requirements of the task.
- Well-Developed Individual Interest––deeper knowledge, positive affect, generates new strategies for work, shows ability to self-regulate and understand their own knowledge.
Self-efficacy is another psychological lens to understanding why girls and non-dominant youth are not well-represented in the fields of STEM, including computer science and engineering. Self-efficacy includes a self-assessment of one’s abilities combined with how long one will persevere within a domain. People with high self-efficacy are more likely to persevere to tackle a difficult task. People who assess themselves as not good at a particular topic often avoid that topic or give up quickly when presented with a challenge. Girls and minority youth often have lower self-efficacy around computer science and engineering (Wilson et al., 2015). For girls and minority youth, improved self-efficacy has been linked to having role models “like oneself” and to pedagogy that includes hands-on, personally relevant, and cooperative work––pedagogical styles not typically seen in many STEM types of courses (Beyer, 2014). Surveys of youth will also include questions to assess their overall self-efficacy and their self-efficacy around computers and engineering.
.
Youth interest in STEM may be difficult to change. An evaluation of 13 programs in Massachusetts to increase youth interest in STEM found that only 5 of the programs produced evidence of increase in youth interest after the program (UMass Donahue Institute, 2011). The successful programs varied widely in their structure, and included an out-of-school high-school biotech internship, a middle school STEM summer camp, an elementary middle and elementary after school math program, an in-school elementary engineering program, and a middle school math program that reached thousands of students with a one-time experience. Almost all provided information on STEM careers, some included collaborative group work, nearly all focused on real-world applications, and all but one engaged youth over a series of several sessions. The program that found a measurable difference in student interest through a single classroom experience was the DIGITS program, in which a STEM professional visited a classroom to talk about their careers (focusing on positive aspects), and lead discussion and activities with the youth. The STEM professionals (“ambassadors”) were trained by the program before the visit, and given a script and guidance for interacting with the youth. Still, it’s possible that a ceiling effect could be in play in some of these programs to increase youth interest: If youth sign up for such optional STEM programs because they already have an interest in STEM, then the program itself may do little to grow their interest.
Projects
Examples of NSF Cyberlearning projects that overlap with topics discussed in this primer.
Computational Thinking
Computer and Information Science
Engineering
In addition, the following projects were reviewed for this Primer.
FIRST Robotics. The mission of FIRST is to “inspire young people to be science and technology leaders and innovators, by engaging them in exciting mentor-based programs that build science, engineering, and technology skills, that inspire innovation, and that foster well-rounded life capabilities including self-efficacy, communication, and leadership.” FIRST is also committed to diversity and inclusion, increasing underrepresented students’ interest in STEM and “developing strategies that will ensure greater access to its programs and reduce inequalities.” FIRST offers 4 programs: LEGO League (middle school), LEGO League Jr. (elementary), Tech Challenge (middle school and high school), and Robotics Competition (high school), each with an accompanying curriculum that is aligned to national science standards. In the high school Robotics Competition (and similarly, in the Tech Challenge), students form teams, build robots, and program them with the help of coaches and mentors over the course of 2-3 months. Each January, a new challenging game is introduced; for example, building a robot that can free-throw a basketball into the basket. The student teams and their mentors work together to solve the challenge, and then teams showcase their work in regional and district competitions. District champions go on to compete in a national competition in April. In 2017, the program reached 85,000 high school students across 3,400 teams in nearly every state in the U.S. Since the program began in 1989, it has engaged more than half a million youth across 59,000 teams creating almost 45,000 robots with the help of 150,000 mentors, and has had substantial impact. Based on its research, FIRST reports that youth who participate in FIRST are more than 2 times as likely to show gains in interest in STEM, and those who participate for more than 1 year show significantly greater gains in STEM knowledge than those who leave after a single year. After participating, 87% plan to take a more challenging math or science course. Alumna are 2.6 times more likely to enroll in an engineering course in their first year of college, and more than 75% are in a STEM field as a student or professional. The impact on girls, in particular, is dramatic.
Technovation. Technovation “invites teams of girls from all over the world to learn and apply the skills needed to solve real-world problems through technology.” The goal of the program is to inspire girls to change the world with technology and pursue computer science as a career. During the intensive 12-week curriculum, small teams of girls work together face-to-face, with a facilitator, ask questions and interact with others in an online forum, and are matched up with female mentors to act as guides and role models. A key part of the program is engaging professional women to serve as mentors and role models to guide, encourage, and help girls overcome challenges, develop self efficacy, and learn to become entrepreneurs. Working with women mentors, the student teams identify a problem in their local community, design and develop a mobile app to address the problem, and then create a business plan and video to pitch their “startup” idea to investors. Since the program began in 2010, it has engaged more than 15,000 girls across 100+ countries and has had substantial impact. Technovation reports that after participating in the program, 78% of students reported more interest in computer science, 70% reported more interest in entrepreneurship, and 67% reported more interest in business leadership. Further, 58% enrolled in subsequent computer science courses and 26% declared a college major in CS, “65x the national rate of 0.4% of female college students majoring in CS”.
Digital Youth Network. Over the years, DYN has offered 5 different initiatives, Digital Youth Divas, Digital Queendom, Robotics, C21, and DYN TV. The DYN team works to develop young people and their technical, creative and analytical skills. They have dedicated spaces and work with Chicago youth over extended periods of time. They have developed diverse supportive environments for youth, and in their Digital Youth Divas (DYD), a two-year, out of school program for middle school girls, they work to have non-dominant girls build their own interests and create their own STEM identities (Pinkard et al., 2017). In their work, they created a digital badging program and say that others should try to reverse engineer their program “because youth everywhere benefit from stronger, connected learning and valuable digital badges.” Badges give program creators a way to better understand what is being learned and learners a way to show what they have learned. Identity development is an explicit part of the program and participants document their interests and identity in their profile as their work; the youth may not realize they are documenting their identity, but that’s what they are doing on the website.
ICT4Me. ICT4Me (formerly BuildIT) is a summer and afterschool program for middle school youth to develop interest, self efficacy, and skills in information technology (IT), and knowledge of possible related careers. The goal of the program is to provide underrepresented youth, and particularly girls, with opportunities to “experience the value of these careers through role models; engage in activities that connect their interests to technology and engineering; and experience success in these activities are powerful motivators for persisting in male-dominated ICT careers and developing their ICT fluency.” The program includes a 6-unit curriculum and assumes, at minimum, that the experience is supported by a manager, a facilitator, visiting IT professionals, and an opportunity (“Family Tech Night”) for students to showcase their work to their family, peers, and the school community. Note that interaction with IT professionals is a key part of the experience: the developers recommend that during each unit, at least two IT professionals should interact with the students, and that “The professionals should be the same gender and race as the youth you work with. Including more people of color and women is a plus.” The site provides tips on how to recruit such professionals who can serve as relatable role models to help students see themselves as someone who could professionals in a variety of careers to encourage youth to consider an ICT career.
Resources
CIRCL Primer: Computational Thinking
CIRCL Spotlight: Principled Assessment of Computational Thinking
CIRCL Webinar Series: Computational Thinking for Teachers & Parents
Girls, Equity, and STEM in Informal Learning Settings: A Review of Literature
Readings
References and key readings documenting the thinking behind the concept, important milestones in the work, foundational examples to build from, and summaries along the way.
Aschbacher, P.R., Li, E., & Roth, E. J. (2010). Is Science Me? High School Students’ Identities, Participation and Aspirations in Science, Engineering, and Medicine. Journal of Research in Science Teaching, 47(5), 564-582.
Bell, A., Chetty, R., Jaravel, X., Petkova, N., & Van Reenen, J. (2017). Who Becomes an Inventor in America? The Importance of Exposure to Innovation. Retrieve on Dec. 7, 2017 from http://www.equality-of-opportunity.org/assets/documents/inventors_paper.pdf
Bell, P., Van Horne, K., & Cheng, B. H. (2017). Special issue: Designing learning environments for equitable disciplinary identification. Journal of the Learning Sciences, 26 (3), 367-375.
Beyer, S. (2014). Why are women underrepresented in Computer Science? Gender differences in stereotypes, self-efficacy, values, and interests and predictors of future CS course-taking and grades. Computer Science Education, 24(2-3), 153-192.
Bienkowski, M. (2018, March). Women in STEM. Presented at SRI Diversity Council Celebrates Women in STEM, SRI International, Menlo Park.
Blikstein, P. (2018). Pre-College computer science education: A Survey of the field. Mountain View, CA: Google LLC. Retrieved from https://goo.gl/gmS1Vm
Burke, R. J., & Mattis, M. C. (Eds.). (2007). Women and minorities in science, technology, engineering, and mathematics: Upping the numbers. Edward Elgar Publishing.
Cheryan, S., Siy, J. O., Vichayapai, M., Drury, B. J., & Kim, S. (2011). Do Female and Male Role Models Who Embody STEM Stereotypes Hinder Women’s Anticipated Success in STEM? Social Psychological and Personality Science, 2(6), 656–664. doi: 10.1177/1948550611405218
Cheryan, S., Master, A., & Meltzoff, A. N. (2015). Cultural stereotypes as gatekeepers: Increasing girls’ interest in computer science and engineering by diversifying stereotypes. Frontiers in psychology, 6, 49.
Ericson, Barbara (2017). AP Data for the United States 1998-2016. Retrieved on May 1, 2018 from http://home.cc.gatech.edu/ice-gt/595
Faber, M., & Unfried, A., & Wiebe, E. N., & Corn, J., & Townsend, L. W., & Collins, T. L. (2013, June). Student Attitudes toward STEM: The Development of Upper Elementary School and Middle/High School Student Surveys. Paper presented at 2013 ASEE Annual Conference & Exposition, Atlanta, Georgia.
Forbes Insights. (2011). Global diversity and inclusion: Fostering innovation through a diverse workforce. Forbes, New York.
Griffith, A. L. (2010). Persistence of women and minorities in STEM field majors: Is it the school that matters? Economics of Education Review, 29(6), 911-922.
Hidi, S., & Renninger, K. A. (2006). The four-phase model of interest development. Educational psychologist, 41(2), 111-127.
Hinton, M. (2016). Eight States Have Fewer Than 10 Girls Take AP Computer Science Exam. Education Week blog post, November 28, 2016.
Kaufman, S. B. (2018). The Role of Luck in Life Success Is Far Greater Than We Realized. Scientific American, March 1, 2018.
Ko, A. (2017). On toys that teach coding. Bits and Behavior blog post, December 19, 2017.
Ko, A. & Davis, K. (2017). Computing mentorship in a software boomtown: Relationships to adolescent interest and beliefs. Proceedings of the ACM International Computing Education Research Conference (ICER ’17). New York: ACM Press.
Margolis, J., & Fisher, A. (2003). Unlocking the clubhouse: Women in computing. MIT press.
Margolis, J., Fisher, A., & Miller, F. (1999). Caring about connections: Gender and computing. IEEE technology and society magazine, 18(4), 13-20.
McGill, M. M., Decker, A., & Settle, A. (2015, July). Does Outreach Impact Choices of Major for Underrepresented Undergraduate Students? In Proceedings of the eleventh annual International Conference on International Computing Education Research (pp. 71-80). ACM.
McGee, E., & Bentley, L. (2017). The Equity Ethic: Black and Latinx College Students Reengineering Their STEM Careers toward Justice. American Journal of Education, 124(1), 1-36.
Medin, D. L., & Bang, M. (2014). Who’s asking?: Native science, western science, and science education. MIT Press.
Pinkard, N., Erete, S., Martin, C. K., & McKinney de Royston, M. (2017) Digital Youth Divas: Exploring Narrative-Driven Curriculum to Spark Middle School Girls’ Interest in Computational Activities. Journal of the Learning Sciences, 26:3, 477-516, DOI: 10.1080/10508406.2017.1307199 . See also a 2015 draft.
Reich, Justin and Mizuko Ito. (2017). From Good Intentions to Real Outcomes: Equity by Design in Learning Technologies. Irvine, CA: Digital Media and Learning Research Hub.
Ruiz, P. (2017). Female Computer Science and Engineering Undergraduates: Reflections on Participation in the Academic Landscape. Dissertation, Pepperdine University Graduate School of Education and Psychology.
Sadler, P.M., Sonnert, G., Hazari. Z., & Tai. R. (2012). Stability and volatility of STEM career interest in high school: A gender study Science Education. 96: 411-427. DOI: 10.1002/sce.21007
UMass Donahue Institute (2011). Increasing student interest in science, technology, engineering, and math (STEM): Massachusetts STEM pipeline fund programs using promising practices. Report prepared for the Massachusetts Department of Higher Education.
Wang, J., Hong, H., Ravitz, J., & Ivory, M. (2015). Gender differences in factors influencing pursuit of computer science and related fields. Proceedings of the 2015 ACM Conference on Innovation and Technology in Computer Science Education, (pp. 117–122). doi: 10.1145/2729094.2742611
Wilson, D. M., Bates, R., Scott, E. P., Painter, S. M., & Shaffer, J. (2015). Differences in self-efficacy among women and minorities in STEM. Journal of Women and Minorities in Science and Engineering, 21(1).
Citation
Primers are developed by small teams of volunteers and licensed under a Creative Commons Attribution 4.0 International License. After citing this primer in your text, consider adding: “Used under a Creative Commons Attribution 4.0 International License (http://creativecommons.org/licenses/by/4.0/).”
Suggested citation:
Fusco, J. & Schank, P. (2018). CIRCL Primer: Broadening Youth Participation in Computer Science and Engineering. In CIRCL Primer Series. Retrieved from http://circlcenter.org/broadening-participation-cs-engineering
Special thanks to Sherry Hsi and Joyce Malen-Smith for reviewing this primer.